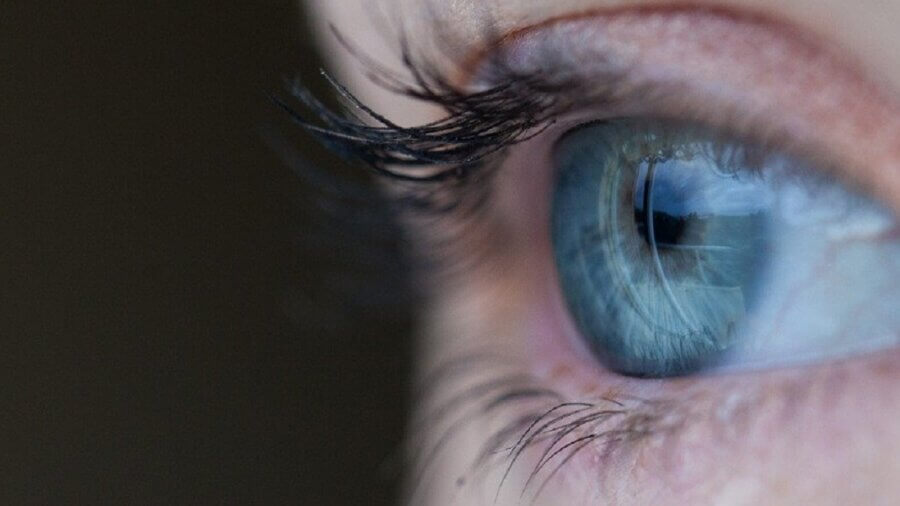
Thanks to gene therapy and optogenetics—a neuroscience game-changer that relies on light to control neurons—a previously blind man can now partially see the world. And he’s doing it with the help of light-sensing algae proteins in his eyes.
With training and a pair of custom goggles, the man could see white stripes on a crosswalk and recognize different objects. His brain’s visual cortex also readjusted to the algae proteins as his new light sensors sparked with activity when his hybrid eyes danced across multiple objects, such as a notebook, staple box, and a bunch of tumblers. His eyesight improved to the point that he could point to an object, reach out and grasp it, and count its numbers.
In other words, his human brain adjusted to visual information from algae light sensors, and was able to make sense of it.
The patient is part of a clinical trial, PIONEER, that combines gene therapy and optogenetics to restore eyesight. As of late 2020, seven patients had been injected with a virus that carries the algae light-sensing gene. Due to Covid-19, only this one patient was able to follow through with the training—but more results will come.
“I hope it will be a major breakthrough,” said Dr. José-Alain Sahel at the University of Pittsburgh and Sorbonne University, who co-led the study with Dr. Botond Roska at the University of Basil.
Why Algae?
The 58-year old man has a condition called retinitis pigmentosa (RP), a genetic disorder that gradually eats away at light-sensing neurons in the retina. Diagnosed four decades ago, his vision had deteriorated so much that he could barely detect flashes of light.
The retina is basically a multi-layered mini-computer that sits at the back of your eyes. When light hits the retina, its top layer translates photons into electrical signals—the language of the brain—thanks to a group of proteins dotted on its surface, called rhodopsins.
These signals are passed through a neural network to the eye-brain liaison, ganglion cells. These guys receive data from other retinal cells—such as those encoding color—and extend their long “arms” to form the main highway between the eyes and the visual cortex. The brain processes the incoming data and brings it into our consciousness, and voilà, we can make sense of what we’re seeing.
In retinitis pigmentosa, the light-sensitive layer of cells is damaged, but the rest of the retina remains intact. So what if we replace our human light-sensing proteins, rhodopsins, with an alternative?
Enter algae. Depending on the species, algae have an impressive repertoire of light-sensing proteins that guide them to move towards light. Over a decade ago, a Stanford team had a spark of ingenuity: what if we take the genes encoding these algae proteins, stick them into mammalian brain cells, and give them the superpower to respond to light?
The result is optogenetics, a mind-controlling technique that’s become one of neuroscience’s most popular tools. Here, scientists use genetic engineering to put different types of algae proteins into the brains of mice. They can then activate a neuron with an implanted fiber optic cable by pulsing certain wavelengths of light. These enhanced brain cells react as they would naturally, generating an electrical signal that’s passed down and interpreted by the mouse’s brain.
Sound familiar?
If an algae protein can artificially allow neurons in the brain to translate light into electrical information, why can’t it do the same for damaged eyes?
Hybrid Eyes
For the new study, the team decided to use ChrimsonR, an algae light-sensing protein that activates under amber-colored light. Developed in 2014 by Dr. Ed Boyden at MIT, ChrimsonR is less toxic than other variants, and has since become an optogenetics darling.
“This is the wavelength that has low light toxicity, which is a very important parameter in optogenetics,” said Sahel.
The genes encoding for ChrimsonR were packaged into a viral delivery system and injected into the man’s poorer functioning eye. The team targeted ganglion cells, the nexus between the eyes and the brain.
“The plan was to… transform his remaining [surviving] ganglion cells,” those that are still connected to his visual brain, and “use optogenetics to activate them,” said Sahel.
It took a few months for the foreign proteins to fully express in the patient’s eyes. In the meantime, the team worked on a separate problem: developing a light source that can transform our colorful world into a monochrome picture that ChrimsonR can detect—remember, it only activates with amber light—so that it can relay as much visual information as possible.
Their solution was a pair of goggles armed with a neuromorphic camera that captures images from our visual world and parses any changes in light intensity, pixel by pixel. The camera would then transform the images into a monochrome picture made with amber light, and project it onto the retina. It’s similar to a movie projector lighting up a theater screen.
The patient then went through multiple exercises to regain his natural instinct for moving his eyes. Once he became aware of the direction of his gaze, he was able to shift his eyes to look straight into the light beams projected by the goggles.
First reaction? The lines vibrated.
From there, the patient was tested with multiple objects placed in front of him. Without the goggles, he couldn’t perceive anything. But once the goggles were turned on, he could sense an object and reach out and grasp it. When challenged with a counting task, his treated eye, with goggles on, showed a clear enough picture for him to point his finger at the objects, and count them correctly 63 percent of the time.
The team also made these tests as real-life as possible. Our world has heavily shifting contrasts in lighting, depending on the season, the weather, or your home light bulbs. Here, they showed objects under three different levels of contrast—for example, a light grey that washes over things, similar to an aged printed photo. Even at low contrasts, the patient was able to detect the object with his new algae-enhanced eyes.
Adaptive Brain
It’s already pretty crazy that sticking an algae protein into someone’s eyes can restore some vision. It’s even crazier that the brain can adjust to a foreign sensor and interpret those signals.
As the patient trained to adapt to his new hybrid eyes and the goggles, the team also measured his visual cortex’s activity using EEG— electroencephalography, a swimming-cap-like system that captures the brain’s electrical signals through the scalp. Amazingly, his visual cortex learned to read information coming in from algae sensors. It showed a clear distinction in electrical patterns between whether a tumbler was placed in front of him or not.
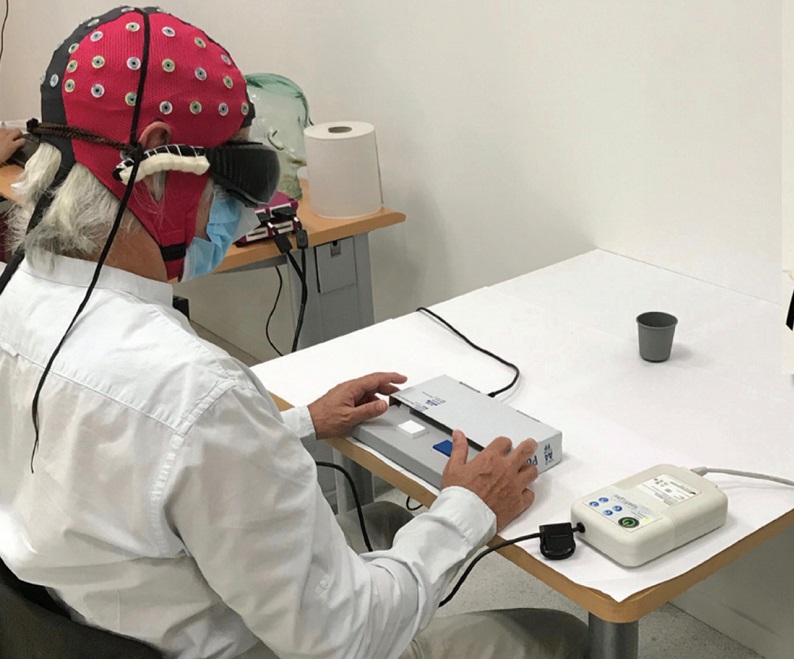
“So far I’ve thought about optogenetics as a tool for scientists, which is where its major impact has been felt. But if it can be directly used in humans, that may open up many frontiers on new kinds of brain-machine interface,” said Boyden, who was not involved in the study.
With many more patients in the pipeline, the team is eager to resume the PIONEER trial. One main question is how much of ChrimsonR to shuttle into the eye without it being toxic. The current trial started with a low dose, then gradually increased—all without notable side effects, and the improvements lasted for at least a year.
For now, the patient doesn’t yet have the visual sharpness to recognize faces, and the lines in his vision are a bit squiggly. He also has tunnel vision, partly because the rest of the retina is harder for the gene therapy to reach. The team next plans to try out the system in everyday life. They’ll also fine-tune the goggles to each volunteer so that they match their normal biological processes and give them the best vision. “We are learning from the patients…they’re telling us what they’re seeing and how they’re using the restored vision,” said Roska.
Image Credit: Free-Photos from Pixabay
* This article was originally published at Singularity Hub
0 Comments