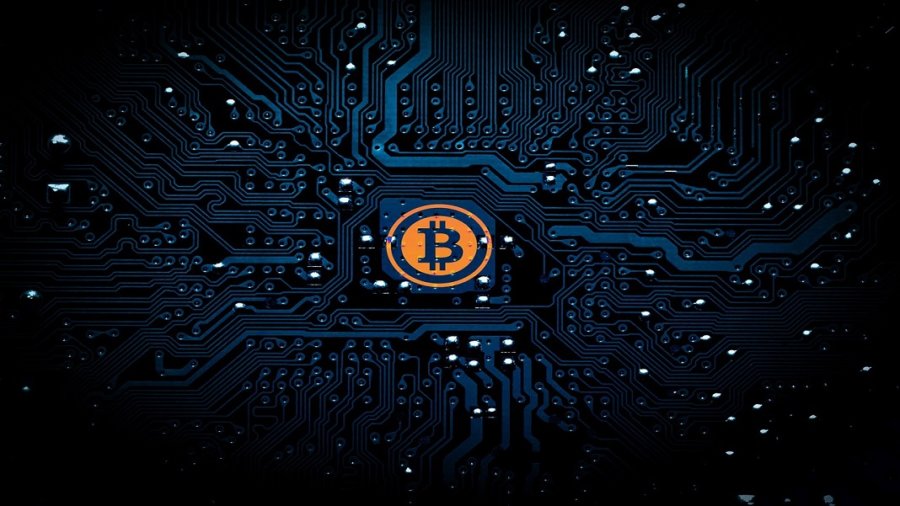
Quantum computers could cause unprecedented disruption in both good and bad ways, from cracking the encryption that secures our data to solving some of chemistry’s most intractable puzzles. New research has given us more clarity about when that might happen.
Modern encryption schemes rely on fiendishly difficult math problems that would take even the largest supercomputers centuries to crack. But the unique capabilities of a quantum computer mean that at sufficient size and power these problems become simple, rendering today’s encryption useless.
That’s a big problem for cybersecurity, and it also poses a major challenge for cryptocurrencies, which use cryptographic keys to secure transactions. If someone could crack the underlying encryption scheme used by Bitcoin, for instance, they would be able to falsify these keys and alter transactions to steal coins or carry out other fraudulent activity.
This would require far larger quantum computers than we have today, but exactly how big is unclear. A new paper in AVS Quantum Science from researchers at British startup Universal Quantum has worked out that it would take a machine with 317 million to 1.9 billion qubits to crack Bitcoin.
The range of qubits is wide because there’s a variable window within which transactions are vulnerable. This is while they’re waiting to be processed, which normally takes between ten minutes to an hour. A quantum computer at the lower end of that scale would be able to pick off some transactions, but only 1.9 billion qubits would guarantee you could target all of them. Sometimes transactions can take as long as a day to go through, in which case the researchers calculated you would need only 13 million.
It’s important to note that these figures relate to a specific kind of quantum computer. Things like how long it takes to carry out a single operation or how much error creeps into calculations can vary significantly depending on the specific type of hardware used to build the quantum computer, and these factors can have a large impact on the number of qubits required.
To get around this, the researchers created a tool that takes these hardware characteristics into account while calculating how big a device was needed for a specific problem. The figures above relate to a machine with one-microsecond operation times, which is typical for the superconducting quantum computers being built by Google and IBM.
Trapped ion devices, favored by Universal Quantum, IonQ, and Honeywell, have operation times closer to 235 microseconds. For those that rely on silicon qubits, times can creep up into the milliseconds, which can significantly increase the number of qubits required.
The researchers also investigated another problem where quantum computers are expected to blow conventional ones out of the water: simulating molecules. The enormous complexity of calculating interactions between even small numbers of particles means most chemistry modeling relies on approximations, and even these require supercomputers. But quantum computers are governed by the same rules as atoms and molecules, and so given enough qubits, should be able to carry out exact simulations within reasonable timeframes.
One promising target for such modeling is the FeMoco molecule that some plants and microorganisms use to fix nitrogen from the air. Understanding how it works could lead to massive efficiency gains in the production of fertilizers, an industry that currently uses two percent of global energy supply.
Conventional computers are incapable of simulating the molecule, but the researchers found that a superconducting device could solve the calculations in 10 days using just 7.5 million qubits. Using the same number of qubits, a trapped ion device would take 2,450 days, which probably isn’t practical, but you can achieve a 10-day turnaround with a 600-million-qubit machine.
The specific design Universal Quantum is targeting does have one trick up its sleeve, though. Superconducting qubits can only talk directly to their neighbors, and any long-range communication requires daisy chains of message-passing interactions that can suck up a lot of operations. In contrast, trapped ion computers are able to physically shuttle their qubits around to allow them to interact directly over much greater distances.
This reduces the number of operations required, which should in turn reduce the number of qubits needed. More importantly, it could open the door to new error-correction schemes that could be considerably more efficient than those used on superconducting devices.
Either way, the research suggests that both cracking Bitcoin and solving nitrogen fixation are likely still a long way off. And more importantly, it shows that scalability is going to be hugely important for quantum computers, particularly for those based on trapped ions, which are likely to need considerably more qubits than their superconducting competitors.
Image Credit: Darwin Laganzon from Pixabay
* This article was originally published at Singularity Hub
0 Comments