What was science fiction is now scientific reality: with a series of targeted electrical zaps to the spinal cord, nine paralyzed people immediately walked again with help from a robot. Five months later, half of the participants no longer needed those zaps to walk.
Does the sentence sound a bit familiar? By themselves, the results—while undeniably impressive and utterly life-changing—may seem like old news. Thanks to improvements in brain implant designs, the last decade saw astonishing progress in restoring mobility to people with paralysis. In 2018, a 29-year-old man walked the length of an entire football field thanks to a few zaps to his spinal cord, after years of paralysis from a snowmobile accident. Last year, spinal cord stimulation helped several people with complete paralysis to stroll around a busy downtown area with a walker and kayak in smooth waters.
There’s no doubt that spinal cord stimulation transformed a once-irreparable injury into one that now can be reversed. But a looming question remains: why does it work?
A new study in Nature just gave us some clues. Building a 3D molecular map of the spinal cord as it recovers from injury, the team found a mysterious group of neurons nestled on its outskirts. They’re peculiar. Normally, these neurons are not required for walking. But in cases of spinal cord injury, after a few electrical jolts they burst with activity, reorganizing into new neural highways that help restore movements.
Pinpointing these neurons isn’t just a scientific curiosity. By understanding how they work, we could tap into their electrical communication and inner molecular workings to develop even more sophisticated treatments for paralysis.
“The amount of hope that it gives to people with spinal-cord injury is incredible,” said Dr. Marc Ruitenberg at the University of Queensland, who was not involved in the study.
To Drs. Kee Wui Huang and Eiman Azim at the Salk Institute for Biological Sciences, who weren’t involved in the study, the results show that tackling spinal cord injury requires embracing multiple angles: improving implant technology—the heart of previous efforts—is just one side of the story. Parsing the neurobiology of recovery is the other critical half.
The new study shows that “high-resolution molecular maps of the nervous system are beginning to provide the latter.”
Bridging the Gap
I like to picture the spinal cord as a buzzing interstate highway. Each section has multiple smaller regional nerve pathways that lead to different portions of the body. As the main information throughline, the spinal cord transmits signals from the brain to the rest of your body. A bad fall, a car accident, or a sports injury can damage that highway. Similar to a roadblock, the electrical traffic sending commands to the muscles—and receiving sensory feedback—can no longer flow through.
But what if we could artificially bridge those road collapses with an implant?
Roughly half a decade ago, scientists began experimenting with a technique called epidural electrical stimulation (EES). The device is made of multiple electrodes and inserted just above the outermost membrane that encapsulates and protects the spinal cord. It acts as an artificial bridge that bypasses the injured spot. A few jolts can activate neurons in the healthy parts of the spinal cord and deliver signals to nearby nerve pathways.
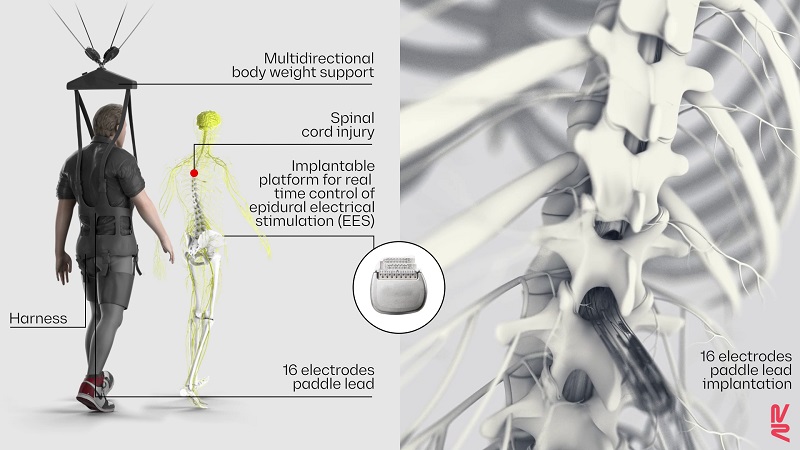
While it’s one of few treatments that has achieved “remarkable changes in performance,” EES has faced multiple setbacks, said Huang and Azim. One was sub-optimal implant design, in that they couldn’t target parts of the spinal cord essential for walking. Another was software powered by algorithms that didn’t stimulate the spinal cord in ways that mimicked its natural electrical pulses. Ironically, those designs may have “disrupted sensory signals that promote recovery,” said Huang and Azim.
From Men to Mice
To get at the heart of how EES helps people recover from paralysis, the new study took an unorthodox approach: they first tested a device and stimulation pattern in patients with paralysis. After confirming their improvement, the team then recreated the treatment in mice with similar injuries to nail down the cells responsible for recovery. The paradigm is a radical departure from typical research proceedings, which start with mice models before moving into humans.
But the team, led by Drs. Grégoire Courtine, a neuroscience professor at EPFL, and Jocelyne Bloch, a neurosurgeon at Lausanne University Hospital (CHUV), have their reasons. Both scientists are no stranger to combating paralysis. Leading the NeuroRestore program, they’ve been at the forefront of engineering spinal cord implants to help patients regain mobility.
In this study, they first stimulated nine people with severe or complete paralysis with EES as part of a clinical trial. Six had some sensation in their legs; the other three had none. The two groups had different hardware implanted, with the first receiving one adapted for pain treatment, and the second developed specifically to stimulate walking. Using a stimulation pattern similar to normal spinal cord signals, the participants immediately improved or regained their ability to walk, with the help of a robot to support their weight. With five more months of training, they gradually learned to support their own weight and could even walk outdoors with help.
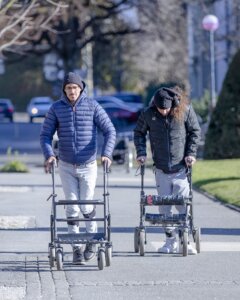
But why? Surprisingly, the team found that EES along with physical rehab decreased the energy needed for parts of the spinal cord that control walking. Rather than engaging all neurons in the spinal cord, EES seems to tailor to just a select group of neurons—those critical to helping patients walk again.
A Molecular Map of Recovery
What are these mysterious neurons?
Digging deeper, the team reran the treatment in mice with paralysis (and yes, it included a custom-made mouse-sized robot to help support their body weight.) Similar to humans, the mice immediately regained their ability to walk with EES turned on.
As they recovered, the team took samples from the spinal cord and sequenced genes in over 80,000 individual cells from 24 mice to see which genes were activated. Location was key: the survey mapped the genes based on each cell’s location in the spinal cord, which together formed the first molecular map of recovery.
You might be thinking it’s a behemoth of a database. Luckily, the team had previously developed a machine-learning algorithm that helps analyze the data. The crux was to match up the gene-expression profiles to certain cells in different biological situations. One particular population of cells called V2a stood out. These neurons were embedded in the region of the spinal cord that’s especially important for walking, and though they weren’t required for walking before injury, seemed to surge with activity after EES.
V2a cells are powerful gatekeepers to spinal cord recovery. In subsequent tests, lowering their activity using optogenetics—a way of controlling neurons with light—also dampened spinal cord recovery.
It shows that “certain types of spinal cord neurons that have lost their inputs from the brain after injury can be ‘reawakened’ or repurposed to restore movement if they are given the appropriate combination of stimulation and rehabilitation,” said Huang and Azim.
V2a cells are hardly a silver bullet for treating spinal cord injuries and paralysis. The study found numerous other neurons—with diverse genetic signatures—that activate with EES. How the brain bypasses spinal cord injury to rebuild their connection is an even more profound mystery. Whether the same neurons help with restoring other everyday bodily needs—bladder and bowel control, for example—is still unknown, but next on the team’s list to study. To that end, the lead author has launched a startup called ONWARD to start a new trial in the next two years.
Image Credit: geralt / 23803 images
* This article was originally published at Singularity Hub
0 Comments